You probably understand what DNA and RNA are. Perhaps, you might have read our article on what genetic materials are, but do you know what they are responsible for? You may also have read our article on what proteins are, but are you aware of how they are made? Today, we will be discussing the central dogma of molecular biology and how it affects protein synthesis.
The Central Dogma of Molecular Biology
The central dogma of molecular biology describes the unilateral flow of genetic information from DNA to mRNA via transcription and mRNA to proteins via translation. To simplify this, it represents the one-way movement of genetic information. This is first achieved by translating DNA into mRNA, and the mRNA is then translated into proteins by ribosomes.
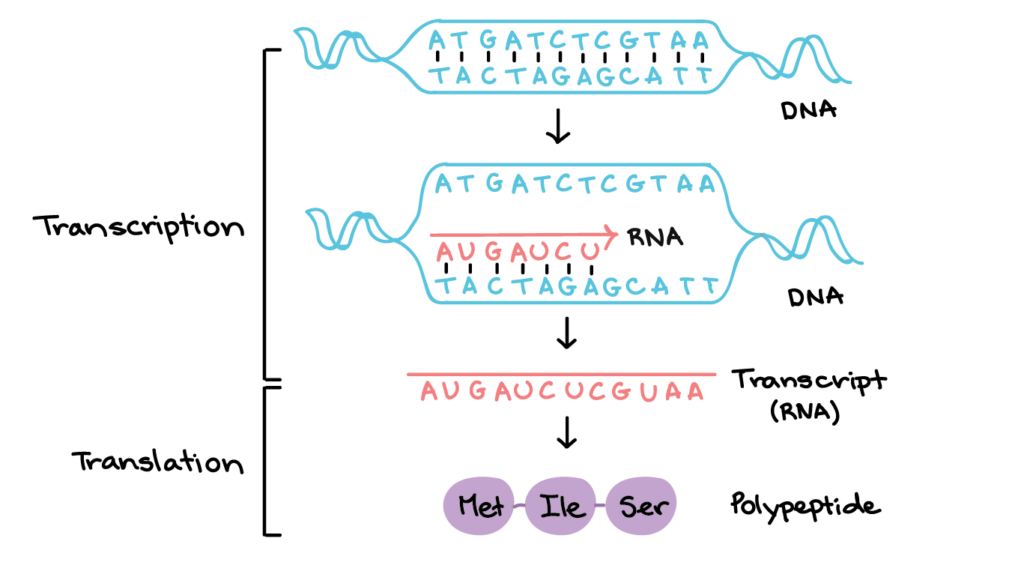
We might also want to talk about what exactly genes are briefly. Well, genes are specific sequences of DNA nucleotides coding for a particular RNA or protein. With that, we can now talk more about transcription
Transcription
Transcription is where DNA nucleotides on a gene’s template strand are replicated onto a complementary mRNA sequence of bases. As DNA is double-stranded, only one strand will serve as the template strand. A transcription unit consists of the promoter, the gene, and the terminator.
Initiation
In prokaryotes, RNA polymerase contains the sigma factor. The sigma factor recognises the promoter and allows the RNA polymerase to bind to it. The RNA polymerase then unwinds the DNA double helix by breaking hydrogen bonds between complementary base pairs, elongating the mRNA. The sigma factor is released when ten or so mRNA nucleotides are synthesised, and the RNA can then continue transcription.
In eukaryotes, general transcription factors recognise the promoter. They then bind to the TATA box at the promoter before recruiting RNA polymerase. RNA polymerase is then positioned on top of the promoter to form the transcription initiation complex. The DNA double helix is then unwound, and transcription is initiated.
Elongation
RNA polymerase catalyses the formation of phosphodiester bonds between mRNA nucleotides that are next to each other. It moves along the template strand in the 3’ to 5’ direction. However, this is not to be confused with the mRNA strand, which elongates in the 5’ to 3’ direction. Free ribonucleoside triphosphates are joint to the template strand via complementary base pairing.
As the mRNA strand is elongated, it peels away from the template strand. DNA is unwound before the transcription bubble and is rewound after transcription.
Termination
In prokaryotes, RNA polymerase transcribes the terminator. As the terminator contains self-complementary sequences, the mRNA forms a hairpin loop due to complementary base pairing. RNA polymerase is then dissociated, and transcription ends.
In eukaryotes, the terminator contains a polyadenylation signal. When transcribed, the RNA transcript is cleaved around 10 to 35 nucleotides downstream of the signal. The RNA transcript, now known as pre-mRNA, undergoes post-transcriptional modifications.
Post-transcription modifications
After transcription is terminated, pre-mRNA undergoes modifications to form mature mRNA. This only occurs in eukaryotes as prokaryotic mRNA lack introns. We will talk more about introns under splicing.
5’ capping
5’ capping occurs when 7-methylguanosine is added to the 5’ end of pre-mRNA. This addition is catalysed by mRNA guanylyltransferase. The 5’ cap prevents the mRNA from degradation and helps export it from the nucleus to the cytoplasm, and it also helps the mRNA bind to ribosomes to be translated.
3’ polyadenylation
3’ polyadenylation occurs when a poly-A tail is added to the 3’ end of the pre-mRNA. This addition is catalysed by poly (A) polymerase. Like the 5’ cap, it prevents mRNA from degradation and helps export the mRNA to the cytoplasm. 3’ polyadenylation also stabilises the mRNA to be used for as many rounds of translation as possible.
Splicing
Splicing occurs when introns on the pre-mRNA are excised and when exons are spliced. As eukaryotic genes are sections called introns and exons, this is transcribed into the pre-mRNA.
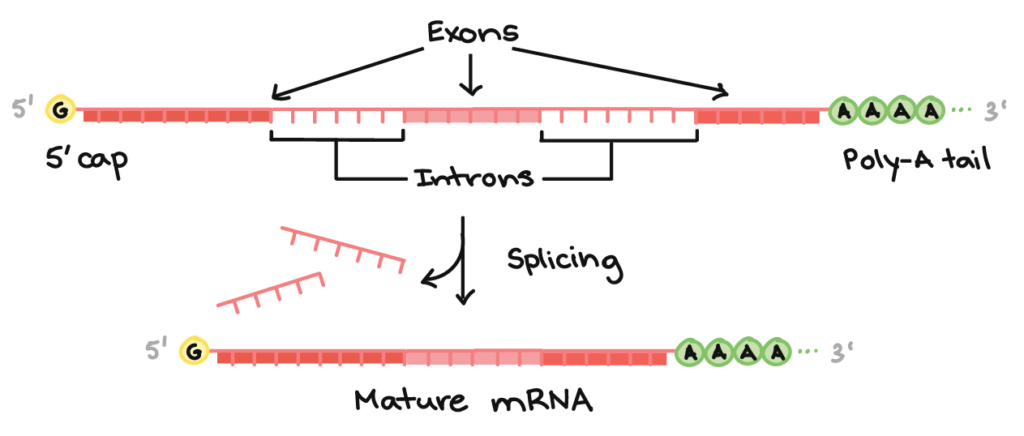
Introns play a part in the alternative splicing of the mRNA, allowing the mRNA to produce multiple protein products, allowing the organism to hold fewer genes. This is done based on the different combinations of introns that are spliced.
Genetic code
The genetic code is the encoding of genetic information in the form of codons. Each codon is made up of three mRNA nucleotides and codes for one amino acid. There are four nucleotide bases and twenty amino acids; three consecutive bases can form 64 different codons. There are three stop codons – UGA, UAA, UAG. There is also a start codon, AUG, which codes for the amino acid methionine. The start codon defines the start point where the codons are to be read.
The genetic code is universal throughout all organisms, and more than one codon can code for an identical amino acid. However, each codon can only code for one amino acid, making the genetic code degenerate but unambiguous. Ultimately, the genetic code determines the sequence of amino acids that make up the protein.
Translation
Now, here is where proteins are made. Before we begin, I must say that this is not easy to understand, so it will be good to familiarise yourselves with details on ribosomes, proteins, and RNA.
Amino acids form aminoacyl-tRNA when attached to tRNA at ribosomes. They then form covalent bonds at the amino acid binding site of tRNAs. This is known as amino acid activation.
Initiation
In prokaryotes, the mRNA binding site of the small ribosomal subunit binds to the Shine-Dalgarno sequence. The sequence is found near the 5′ end of the mRNA before the start codon. The start codon marks the future peptidyl-tRNA site (P site). Initiator tRNA carries formyl-methionine to allow the following amino acid to be added to the carboxyl end. This ensures that the polypeptide chain is elongated from the N-terminus to the C-terminus.
The initiator tRNA binds to the start codon via complementary base pairing with the anticodon. The translation-initiation complex is then formed when the large ribosomal subunit binds.
In eukaryotes, the initiator tRNA carries methionine, an amino acid. Initiator tRNA binds to the small ribosomal subunit. The small subunit then attaches to the mRNA’s 5′ untranslated region (5′ UTR) at the mRNA binding site, and it moves downstream until it reaches the start codon.
The initiator tRNA’s anticodon then binds to the start codon via complementary base pairing. The translation-initiation complex is then formed when the large ribosomal subunit binds.
Elongation
The polypeptide chain is elongated via the elongation cycle.
Aminoacyl-tRNA of the subsequent codon first binds to the mRNA at the aminoacyl-tRNA site (A site) via complementary base pairing. The amino acids in the P site and the A site then form peptide bonds between each other. This is catalysed by peptidyl transferase in the large ribosomal subunit.
The ribosome then moves a codon down the template in the 5′ to 3′ direction. The free tRNA from the P site shifts to the exit site (E site), effectively releasing it. The peptidyl-tRNA then moves from the A site to the P site, and a new aminoacyl-tRNA can then enter the now-empty A site. Elongation continues, and the cycle repeats.
Termination
When the ribosomes reach the stop codon, the A site takes in a release factor instead of an aminoacyl-tRNA. The release factor then hydrolyses the bond between the polypeptide chain and the P site’s tRNA. The ribosomal subunits then dissociate, and the protein is released.
Post-translation modifications
After translation, modifications are made to proteins to control their function and regulate the number of proteins. Biochemical modifications can be done by adding chemical groups to proteins, enabling the protein to be functional. Some proteins may also need to be cleaved to be folded, and this is done via proteolytic cleavage.
For proteins that are no longer in use, they are degraded to recycle raw materials. In eukaryotes, this is done via ubiquitinylation. Ubiquitin is added to the N-terminus of the protein, marking it for degradation by proteasomes.
Conclusion
Now that you have understood the process behind how DNA is transcribed into mRNA, which is then translated into proteins, you can better understand how this gives rise to different functions of proteins in your body. Of course, transcription and translation are difficult to understand on their own, so you should probably do some research and figure out which aspects of it you are unsure of.
Add comment